We all know that EV batteries generate heat, which is managed through liquid-cooled or air-cooled systems. But when a battery overheats beyond its limit, it can trigger a phenomenon called thermal runaway.
This occurs when a battery’s internal temperature rises uncontrollably, causing rapid heat generation in a very short time. The temperature increases exponentially and releases flammable gases, which in worst-case scenarios can lead to fires or explosions, endangering both the vehicle and its passengers. Thermal runaway is a serious safety concern that shakes consumer trust in EVs.
Cutting-edge technologies are being developed to prevent thermal runaway and ensure battery safety. Real-world incidents have highlighted the risks, prompting global efforts to study and implement preventive solutions. This post is for EV owners, enthusiasts, and Engineers, researchers, professionals looking to understand safety challenges in EV batteries.
What Is Thermal Runaway?
Thermal runaway occurs when an EV battery’s internal temperature rises uncontrollably, triggering a chain reaction that generates even more heat.
• The Science Behind It:
• Heat causes the battery’s electrolyte to break down, releasing gases.
• The buildup of pressure and heat can lead to fires or explosions.
• Simple Analogy:
• “Think of thermal runaway like a snowball rolling downhill—it starts small but quickly becomes an unstoppable force.”
Section 2: Causes of Thermal Runaway in EV Batteries
• Key Triggers:
1. Overcharging:
• Causes excessive heat buildup in battery cells.
2. Physical Damage:
• Crushed or punctured batteries can short-circuit, leading to heat generation.
3. Manufacturing Defects:
• Poor quality control can result in internal short circuits.
4. Extreme Temperatures:
• Both high and low temperatures stress battery cells.
5. Internal Resistance:
• Over time, aging batteries develop higher resistance, leading to more heat generation.
Section 3: Real-Life Examples of Thermal Runaway
• Notable Incidents:
1. Chevrolet Bolt Recall (2021):
• GM recalled thousands of EVs after multiple reports of battery fires linked to thermal runaway.
2. Tesla Model S Incident (2018):
• A parked Tesla caught fire due to a damaged battery pack.
3. Cargo Ship Fire (2022):
• Electric vehicles on a cargo ship caught fire, with thermal runaway suspected as the cause.
• What We Learn from These Examples:
• Highlight the need for robust thermal management systems and battery safety standards.
Section 4: How Is Thermal Runaway Prevented?
1. Battery Thermal Management Systems (BTMS):
• What They Do:
• Monitor and regulate the temperature of EV batteries.
• Types of BTMS:
1. Liquid Cooling:
• Uses coolant to absorb and dissipate heat (used by Tesla).
2. Air Cooling:
• Fans or vents to regulate temperature (common in budget EVs).
2. Advanced Safety Features in Battery Packs:
• Fireproof Barriers:
• Separate battery cells to prevent the spread of heat.
• Ventilation Systems:
• Release pressure and gases safely to avoid explosions.
• Thermal Sensors:
• Detect overheating and shut down the system to prevent escalation.
3. Battery Design Improvements:
• Solid-State Batteries:
• Replace flammable liquid electrolytes with solid ones, reducing fire risks.
• Cylindrical Cells (e.g., Tesla’s 4680):
• Uniform shape and better thermal stability compared to pouch cells.
4. Smart Battery Management Systems (BMS):
• Real-time monitoring of voltage, temperature, and charge levels.
• Balances cells to prevent localized overheating.
Section 5: Practical Tips for EV Owners
• How to Minimize Risks:
1. Avoid charging your EV in extreme heat or cold.
2. Don’t leave your EV plugged in after it’s fully charged.
3. Use manufacturer-recommended chargers to prevent overcharging.
4. Monitor recalls or software updates for your EV model.
• Signs of Battery Issues to Watch For:
• Unusual heat or swelling in the battery.
• Sudden drops in range or performance.
Section 6: Future Innovations in Preventing Thermal Runaway
• Emerging Technologies:
1. Solid-State Batteries:
• Safer designs with reduced risk of thermal runaway.
2. AI-Powered Battery Monitoring:
• Predicts and prevents overheating with advanced algorithms.
3. Nano-Coatings:
• Prevent electrolyte breakdown and slow heat generation.
• Industry Collaboration:
• EV manufacturers and battery companies are working together to establish global safety standards.
Conclusion: A Safer Future for EV Batteries
• Summarize the importance of understanding thermal runaway and how it’s being addressed with technology and safety measures.
• Encourage readers to adopt practical safety tips and stay informed about their EV’s battery system.
• End with a call-to-action:
“Have questions about EV battery safety? Explore our guides on battery management and thermal efficiency!”
3. Writing Style and Tone
• Tone: Informative yet accessible to appeal to a wide range of readers.
• Use examples and analogies:
• “Think of a battery management system as a firefighter—it detects heat early and stops a potential blaze in its tracks.”
4. Suggested Visuals and Graphics
1. Infographic: The process of thermal runaway (heat generation → chain reaction → fire/explosion).
2. Diagram: Components of a thermal management system in EV batteries.
3. Real-World Image: Examples of EV battery cooling systems (e.g., Tesla’s liquid cooling system).
4. Comparison Table: Traditional vs solid-state batteries in preventing thermal runaway.
5. SEO Optimization Strategy
Focus Keyword:
“Thermal runaway in EV batteries”
Supporting Keywords:
• Preventing EV battery fires
• Thermal management in EVs
• Causes of battery overheating
• EV battery cooling systems
Meta Description:
“Learn about thermal runaway in EV batteries: what causes it, how it’s prevented, and real-life examples. Discover how thermal management and safety innovations protect EVs from overheating risks.”
Alt Text for Feature Image:
“Diagram of thermal runaway in EV batteries showing heat buildup and prevention systems.”
6. Blog Length
• Target Word Count: 1,800–2,200 words to thoroughly explain technical concepts and solutions.
By following this strategy, the blog will provide a clear, engaging, and SEO-optimized explanation of thermal runaway, its risks, and the safety measures being adopted to make EV batteries safer for everyone.
The transition to electric vehicles (EVs) is a cornerstone of the global shift towards sustainable transportation. At the heart of every EV lies its battery, the critical component that stores electrical energy for propulsion. The type of cell used in an EV battery plays a pivotal role in determining the vehicle’s range, performance, and longevity. This blog post delves into the various types of EV cells, shedding light on their characteristics, advantages, and limitations.
Lithium-Ion Cells
Lithium-ion cells are the most prevalent in the current generation of electric vehicles. Renowned for their high energy density, which allows for longer ranges on a single charge, these cells have become the industry standard. They are also relatively lightweight, contributing to overall vehicle efficiency. However, lithium-ion cells require sophisticated management systems to ensure safety and longevity, as they can be prone to overheating.
Advantages:
- High energy density
- Lightweight
- Rechargeable
Limitations:
- Higher cost
- Thermal sensitivity requiring advanced cooling systems
Nickel-Metal Hydride (NiMH) Cells
Before lithium-ion cells dominated the scene, nickel-metal hydride cells were the go-to for early electric and hybrid vehicles. While they offer a decent energy density and a lower risk of thermal runaway compared to lithium-ion, their heavier weight and lower efficiency at higher temperatures make them less favorable for pure EV applications today.
Advantages:
- Good energy density
- More stable at high temperatures than lithium-ion
Limitations:
- Heavier than lithium-ion
- Less efficient in high-temperature environments
Solid-State Cells
Solid-state batteries represent the next frontier in EV cell technology. Unlike conventional lithium-ion cells that use liquid electrolytes, solid-state batteries use a solid electrolyte. This innovation promises to significantly increase energy density, offering the potential for longer ranges and reduced charging times. Moreover, solid-state cells are believed to be safer, with a lower risk of leaking or catching fire.
Advantages:
- Higher energy density
- Potentially safer than liquid electrolyte cells
- Longer lifespan
Limitations:
- Currently expensive and challenging to mass-produce
- Technology is still under development
Lithium Iron Phosphate (LiFePO4) Cells
Lithium iron phosphate cells are gaining traction for their safety and stability advantages over traditional lithium-ion chemistry. While they offer a lower energy density, they compensate with a much longer cycle life and better thermal stability, reducing the risk of overheating and improving overall battery longevity.
Advantages:
- Excellent thermal stability and safety
- Long cycle life
Limitations:
- Lower energy density compared to standard lithium-ion cells
Ultra-Capacitors
While not traditional batteries, ultra-capacitors (also known as supercapacitors) are worth mentioning for their role in some EV applications. These devices store less energy than batteries but can charge and discharge very quickly. They are ideal for applications requiring rapid bursts of power, such as regenerative braking systems in EVs.
Advantages:
- Rapid charging and discharging
- Long lifespan
Limitations:
- Low energy density
- Currently more of a complementary technology to batteries
Comparison & Graphical Analysis:
Type | Energy Density (Wh/kg) | Power Density (W/kg) | Cycle Life | Safety |
---|---|---|---|---|
Lithium-Ion Cells | 265 | 3000 | 1500 cycles | Moderate |
NiMH Cells | 120 | 1000 | 3000 cycles | High |
Solid-State Cells | 500 | 6000 | 10,000 cycles | Very High |
LiFePO4 Cells | 120 | 2000 | 3000 cycles | High |
Ultra-Capacitors | 10 | 20,000 | 1,000,000 cycles | Very High |
Insights
- Solid-State Cells offer a superior balance of high energy density and significant power density, coupled with excellent safety and long cycle life, making them promising for future applications where energy efficiency and safety are paramount.
- Lithium-Ion Cells remain the choice for applications requiring a good balance of energy density and power density, though they have moderate safety concerns.
- Ultra-Capacitors, while having low energy density, provide extremely high power density and an exceptionally long cycle life, ideal for applications needing quick energy bursts without the degradation concerns typical of chemical batteries.
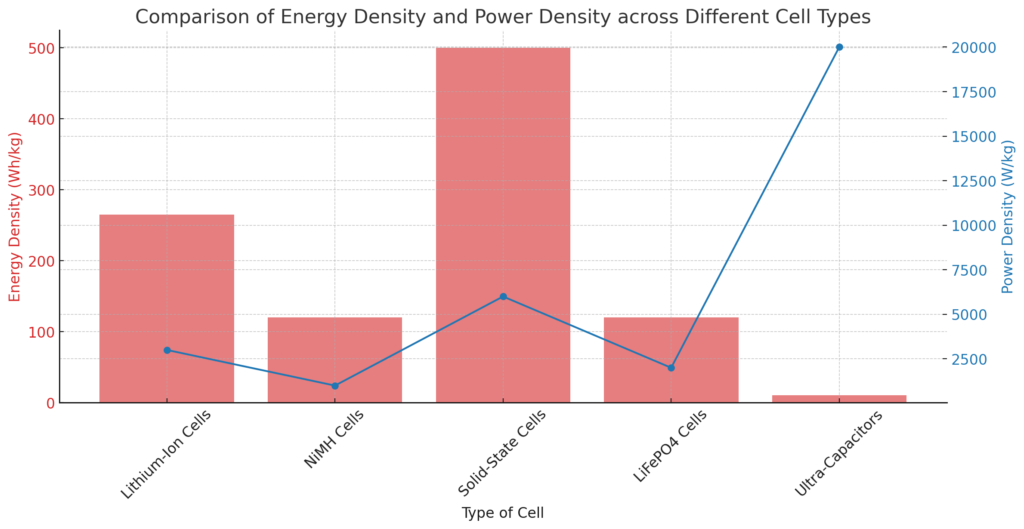
This data helps in selecting the right technology based on specific application needs, considering factors such as required lifespan, safety, energy storage capacity, and power delivery speed.
The Future of EV Cells
As the EV market evolves, the development of battery cell technology remains a hotbed of innovation. The race is on to find the optimal balance between energy density, safety, cost, and environmental impact. Solid-state technology, in particular, holds promise for the future, with the potential to significantly enhance EV performance and range. Meanwhile, advances in recycling and material recovery are crucial for addressing the sustainability concerns associated with battery production and disposal.
The choice of EV cell type is more than just a technical decision; it reflects a broader commitment to efficiency, safety, and sustainability in the quest for cleaner transportation solutions. As research and development continue to push the boundaries of what’s possible, the future of electric vehicles looks brighter—and more electrified—than ever.