In the ever-evolving landscape of electric vehicle (EV) technology, the design and thermal management of motor rotors bearing permanent magnets represent a crucial challenge. As an industry veteran with years of experience, I’ve seen firsthand how the efficiency and longevity of electric motors hinge on managing rotor temperature. High temperatures can degrade permanent magnets and impact motor performance. This blog post delves into the causes of high rotor temperatures, design considerations, strategies for thermal management, and the effects of different waveform types on rotor heat generation.
1. Causes of High Rotor Temperature
High rotor temperature in electric motors can be caused by several factors, each affecting the motor’s efficiency, performance, and lifespan. Understanding these causes is crucial for maintaining optimal motor operation and preventing premature failure.
Eddy Currents
One of the primary sources of heat in rotors with permanent magnets is eddy currents. These are loops of electrical current induced within the rotor due to the changing magnetic field during operation. Although these currents are an inherent part of motor operation, their heating effect can be substantial, leading to increased rotor temperatures.
Mechanical Losses
Friction within the motor bearings and air resistance also contribute to mechanical losses, generating heat as the rotor spins. While less significant than eddy currents, they still play a role in the overall thermal profile of the motor.
Misalignment, bearing wear, and other mechanical issues can also lead to increased friction and, consequently, heat. Ensuring that all mechanical parts of the motor are in good condition and properly aligned can help mitigate this source of heat
Inadequate Cooling
Proper heat dissipation is essential for maintaining a motor’s temperature within safe limits. Lack of adequate cooling or ventilation can lead to significant temperature rises, causing overheating. Ensuring that the motor has enough space and unobstructed airflow for cooling is critical. (source)
Overloading
Operating a motor beyond its rated capacity leads to excessive heat generation. This overloading can be due to demanding more power than the motor is designed to handle or due to environmental factors that reduce its effective capacity, such as high ambient temperatures or altitudes where thinner air provides less cooling. (source)
Electrical Imbalances
Imbalances in the three-phase power supply can lead to uneven current distribution among the motor windings. This imbalance often results in higher current in one or more phases, increasing the heat generated within the motor. Regular maintenance and checks can help identify and correct these imbalances. (source)
Voltage Irregularities
Incorrect voltage supply, either too high or too low, can force the motor to operate inefficiently. This inefficiency typically manifests as heat due to increased electrical resistance in the motor’s components when they are forced to operate outside their optimal voltage range. (source)
Phase Imbalance
In three-phase motors, an imbalance among the phases can cause excessive currents that produce additional heat. This kind of imbalance can severely impact motor performance and increase temperature significantly. (source)
To mitigate these risks, regular monitoring of motor temperature, proper installation, and adherence to operational guidelines are essential. Preventative maintenance, such as regular checks and balances on the motor’s electrical and mechanical systems, is also crucial to ensure the motor operates within its thermal limits, thereby extending its lifespan and maintaining its efficiency.
2. Design Criteria for Thermal Management
When designing rotors, several criteria must be considered to ensure effective thermal management:
Material Selection
The choice of materials for the rotor and permanent magnets is critical. Materials with high thermal conductivity can help dissipate heat more effectively. Additionally, the magnetic materials must be able to withstand high temperatures without losing their magnetic properties.
Geometry and Configuration
The shape and configuration of the rotor influence its thermal performance. Designers aim for geometries that minimize eddy current generation while facilitating heat dissipation. This often involves optimizing the thickness of rotor laminations and the placement of permanent magnets.
3. Strategies for Thermal Management
Active Cooling Systems
Incorporating cooling systems, such as liquid cooling jackets around the motor or air cooling with strategically placed vents, can significantly reduce rotor temperature. These systems directly remove heat from the motor, protecting the permanent magnets and other critical components.
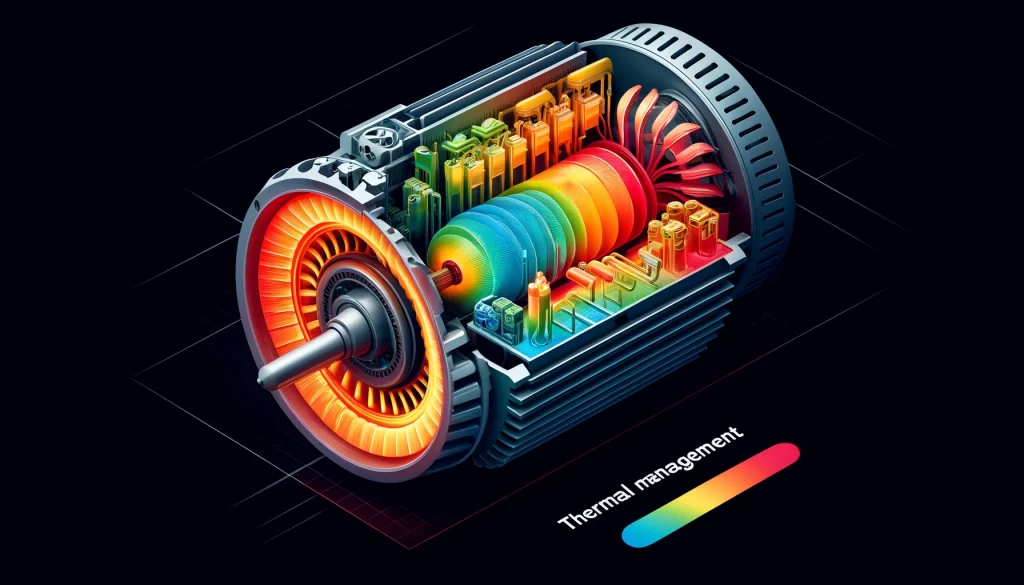
Lamination and Segmentation
Segmenting the permanent magnets and using thin laminations for the rotor core can reduce eddy current losses. This approach limits the path available for eddy currents, minimizing their heating effect.
4. Rotor Design Considerations
Eddy Current Reduction
Designers focus on minimizing eddy currents through material selection and rotor geometry. Advanced simulation tools allow for the precise modeling of eddy currents and their impact on rotor temperature, enabling optimized designs.
Heat Dissipation Pathways
Creating effective pathways for heat to leave the rotor is paramount. This involves not just the rotor design itself but also how the rotor interacts with the motor’s overall cooling system.
Durability Under Thermal Stress
The rotor must be designed to withstand thermal expansion and the stress associated with repeated heating and cooling cycles. This requires materials and designs that maintain structural integrity and magnetic properties over a wide temperature range.
5. Waveform Impact on Rotor Heat
Trapezoidal Waveform
Trapezoidal waveforms are often used in brushless DC motors. While efficient, they can lead to uneven magnetic fields that exacerbate eddy current generation and, consequently, heat within the rotor.
Sinusoidal Waveform
Sinusoidal waveforms, common in AC motors, provide a more uniform magnetic field, potentially reducing eddy current losses. However, the design must carefully balance the waveform characteristics with the motor’s magnetic design to optimize efficiency and minimize heat generation.
Conclusion
The thermal management of rotors in electric motors is a complex, multifaceted challenge that demands a thorough understanding of materials science, electromagnetism, and fluid dynamics. As the industry pushes towards higher efficiency and power density, the role of innovative rotor designs and advanced cooling strategies becomes increasingly critical. By meticulously addressing the causes of heat generation and employing strategic design and cooling methods, we can significantly enhance the performance and reliability of electric motors, paving the way for the next generation of electric vehicles.
Navigating the intricacies of rotor design and thermal management requires a blend of theoretical knowledge and practical experience. As we continue to innovate and refine electric motor technologies, the lessons learned from each design iteration contribute to the broader knowledge base, driving the industry forward towards more sustainable and efficient transportation solutions.