Like the engine is the heart of an internal combustion engine (ICE) vehicle, the EV battery is the heart of an electric vehicle, determining everything from its range to its price. But what goes into making these batteries, and where is this technology headed?
Batteries, like fuel in ICE vehicles, store chemical energy and produce electricity to power EVs. They also play a key role in the performance and stability of the vehicle. As EVs transform the mobility industry with cleaner and more sustainable solutions, advanced batteries and energy storage systems are at the core of this shift.
This article explores the types, components, and evolving technologies of EV batteries, making it a must-read for tech enthusiasts, professionals in the EV and battery industry, and traditional automotive experts looking to understand this critical energy system.
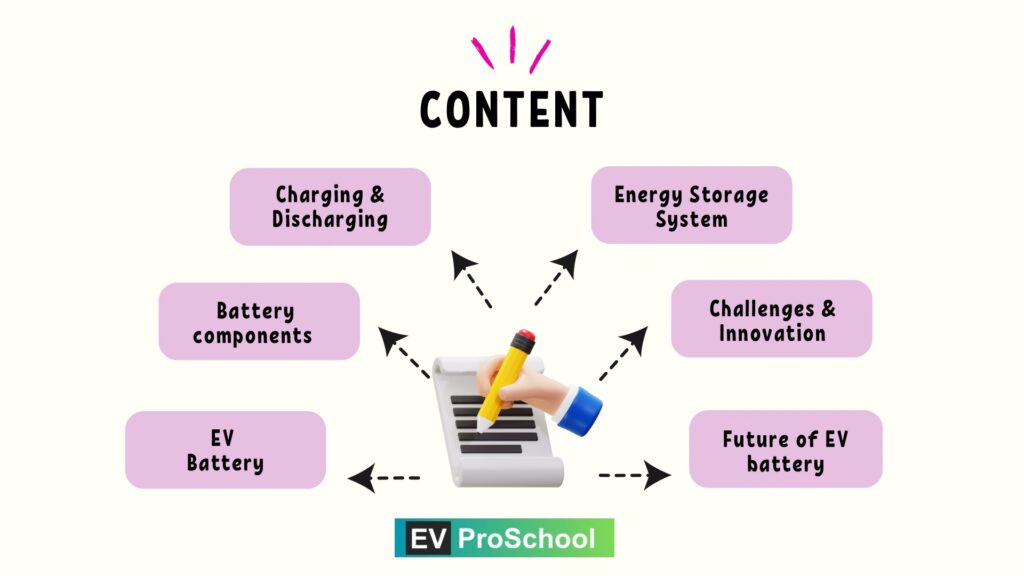
1. EV Battery & Energy Storage Systems
Role of EV Batteries
- EV batteries are the primary energy source, similar to how the Sun powers the Earth.
- They provide electrical energy to power motors and auxiliary systems in electric vehicles.
- Batteries in EVs act as small energy storage systems, – comparable to larger battery packs used in power grids.
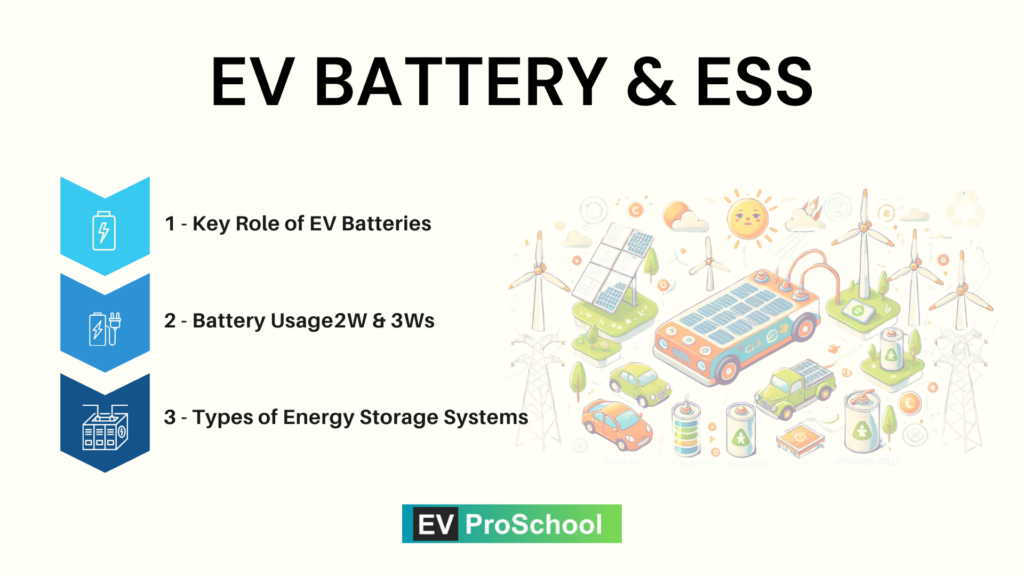
Battery Usage in 2W & 3Ws
- Low-voltage batteries (<60V, e.g., 48V) power small motors (2-6 kW) in light EVs.
- A DC-DC converter reduces 48V to 12V to power auxiliary systems like lights, indicators, and small applications, as well as charge a 12V battery if needed.
Types of Energy Storage Systems
- Batteries: Core energy storage units used in EVs and power grids.
- Supercapacitors: Store and release large, sudden bursts of energy where batteries fall short.
- Flywheels and Hydrogen Fuel Cells: Other examples of energy storage systems.
2. Breaking Down EV Battery Components
Battery cells are the smallest units generating electricity, grouped into modules for better safety and thermal management. Modules are then assembled into packs with cooling systems and controlled by the BMS, which acts as the brain of the battery. This layered design ensures reliability, safety, and optimized performance in electric vehicles.
Types of Battery Cells
Cylindrical Cells:
- Shaped like small cylinders (e.g., Tesla’s 4680 cells).
- Popular for EVs due to ease of manufacturing and better thermal management.
- Originated when Elon Musk used laptop batteries for the Tesla Roadster.
Prismatic Cells:
- Rectangular shape, enclosed in metal or plastic.
- Compact design, commonly used in passenger EVs (e.g., BMW i3).
Pouch Cells:
- Flexible and flat, like a soft bag.
- Used in compact EVs for space optimization and lightweight design.
Why Small Cells Are Preferred:
- Thermal Management: Smaller cells dissipate heat better, reducing the risk of overheating.
- Fault Isolation: If one cell fails, the impact is minimal, improving overall safety.
- Design Flexibility: Easier to group into modules and packs based on voltage and capacity needs.
Series and Parallel Connections:
- Parallel: Increases capacity (e.g., 5 cells of 3.7V, 6Ah = 30Ah at 3.7V).
- Series: Increases voltage (e.g., 5 cells of 3.7V, 10Ah = 18.5V at 10Ah).
Modules and Packs:
Modules:
- A collection of cells grouped together for safety and performance.
- If one module overheats, it can be isolated without affecting the entire pack.
- Easier maintenance as damaged modules can be replaced individually.
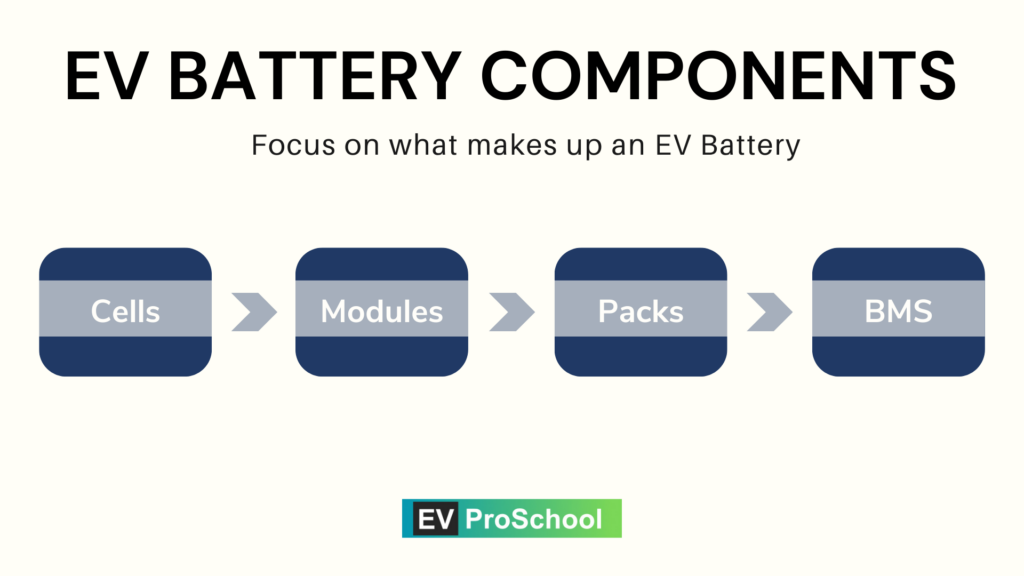
Packs:
- Multiple modules assembled with cooling systems and structural integrity.
- Equipped with numerous temperature sensors (e.g., 7 sensors per module) for real-time monitoring.
- Data from sensors feeds into the Battery Management System (BMS).
Battery Management System (BMS):
Role:
- Monitors temperature, voltage, and performance of each cell and module.
- Ensures balanced charging/discharging to prevent overcharging or undercharging.
- Optimizes battery life and safety.
Functions:
- Generates heat maps and manages thermal conditions.
- Communicates with the vehicle control unit to display state of charge (SOC) and range.
- Independently shuts down the battery pack in case of faults like overheating or short circuits.
3. EV Battery Charging and Discharging Cycles
Battery charging and discharging are fundamental aspects that impact EV performance and range. When an EV is charged, lithium ions move from the cathode to the anode. During discharge (when the vehicle is in use), these ions travel back to the cathode, generating the electric current that powers the motor.
- Charging Rates: EVs can charge at different rates—Level 1 (slow), Level 2 (standard), and Level 3 (fast DC). Each has different impacts on the battery’s longevity and thermal management requirements.
- Depth of Discharge (DoD): The percentage of the battery discharged relative to its total capacity, DoD impacts the cycle life. Partial discharges typically extend battery life compared to complete discharges.
- Fast Charging Implications: While fast charging is convenient, it generates heat, which can degrade the battery over time if not managed properly. Innovations in battery cooling systems help address this issue, allowing for rapid, safe charging.
4. Battery Energy Storage Systems and Their Role
In addition to providing propulsion, EV batteries serve as energy storage systems that can interact with the electric grid. This ability has led to innovations such as vehicle-to-grid (V2G) technology, which allows EVs to return excess power to the grid during high-demand periods. Such applications make EVs not just consumers of energy but potential contributors to a more resilient and sustainable energy ecosystem.
- Vehicle-to-Home (V2H): In addition to supporting the grid, some EVs can provide backup power to homes in case of outages, contributing to energy independence.
- Battery Recycling and Reuse: As EV adoption grows, so does the need for sustainable battery disposal and recycling practices. Companies are exploring ways to repurpose used EV batteries for stationary energy storage or recycle valuable materials to minimize environmental impact.
5. Challenges and Innovations in EV Battery Technology
While EV batteries have made significant advances, several challenges remain:
- Cost and Affordability: EV batteries are one of the most expensive components, making affordability a challenge. However, with increased production and advancements in technology, battery prices continue to decrease.
- Range Anxiety: For EV drivers, range is a critical factor. Battery improvements are gradually addressing this concern by increasing energy density and efficiency.
- Temperature Sensitivity: Batteries perform best within certain temperature ranges. Extreme heat or cold can reduce range and efficiency, making battery thermal management essential.
Innovations are underway to address these challenges. Solid-state batteries, advanced thermal management systems, and AI-driven battery management are just a few of the promising technologies that aim to increase battery life, efficiency, and sustainability.
6. The Future of EV Batteries
The future of EV batteries is promising, with ongoing research and investment. New materials, such as solid electrolytes in solid-state batteries, promise to enhance safety and energy density. Researchers are also exploring “quantum” batteries, which could theoretically store immense amounts of energy in a small space.
Additionally, EV manufacturers are collaborating with energy companies to develop robust charging infrastructure and explore second-life applications for used batteries. Battery recycling, improved raw material sourcing, and green manufacturing processes are essential steps to ensure that the EV revolution remains environmentally sustainable.
Conclusion
EV batteries and energy storage systems are the backbone of electric vehicles, impacting everything from range and performance to charging infrastructure and sustainability. As battery technology continues to advance, EVs are becoming more accessible, efficient, and eco-friendly. Whether through higher energy densities, solid-state advancements, or V2G capabilities, the future of EV batteries holds the potential to revolutionize transportation and contribute to a cleaner, more resilient energy grid.